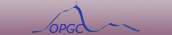 |
|
Recherche |
|
Validation
Analyses of the erupted products (explosive or effusive) in terms of their grain size, componentry, morphology, density, permeability, bubble and crystal contents (and size distributions), as well as their distribution in space and time, allow us to reduce geophysical data, as well as to validate the output and properly constrain the event. In addition, when coupled with chemical data and derived rheological conditions, we can fully define source conditions, as well as apply explosion source, plus plume emission and ascent, models.
When these observations are made at active explosive and effusive volcanic systems provide:
- Ground truth and validation data for remote or geophysical measurements;
- Input for modeling and reduction of geophysical data to allow extraction of meaningful parameters;
- Constraint for, and input into, physical models;
- Correlations between, and integration with, geophysical data to better understand volcanic systems.
Ground truth, input for modeling of geophysical data, constraint for physical models
This data base is designed to be of value to those modeling all aspects of active volcanism, including:
- Magma chamber conditions;
- Conduit dynamics in basaltic and more evolved volcanic systems;
- Ascending, dispersing and collapsing ash plumes and clouds;
- Pyroclastic density current (PDC) dynamics;
- Lava flow emplacement dynamics,
- Reduction of geophysical data sets, and
- Full linking and understanding of system dynamics.
To achieve this, this data base provides values that can be used as boundary conditions and/or input for the models, or to test the validity of theoretical output and hypotheses.
A few examples include:
- Conduit models usually have to assume, or be consistent with, plausible magma viscosities, densities, volatile contents, ascent rates, and exit velocities. Some conduit models also often assume homogeneous conditions, when magma properties likely differ greatly, especially towards the free-surface in a basaltic system. These assumptions all need support and validation.
- Plume ascent, fall out and ballistic dispersion models require input of appropriate values for mass flux, at-vent velocity, plume density, particle size and mass.
- Lava flow emplacement models require input of (or need to operate within) appropriate rheological limits, as defined by internal temperature, crystallinity and vesicularity (number, shape and size). Output also need to be consistent with real velocities, effusion rates, run out distances, dimensions and coverage areas.
- PDC dispersion and emplacement models require input that can be derived from the sedimentalogical features of the deposits, grain size, maximum coverage, temperature, flow direction, presence of obstacles and roughness. Output also needs to be consistent with real velocities, magma discharge rates, run out distances, dimensions and coverage areas.
- Reduction of geophysical data sets to extract higher level parameters requires input of data that can be provided by sample analysis. While conversion of thermal data to dense rock mass and mass fluxes, for example, requires knowledge of the particle density and/or vesicularity, conversion of Doppler radar data to mass flux requires knowledge of the particle size distribution within the plume.
Correlations between, and integration with, geophysical data to better understand volcanic systems: an example
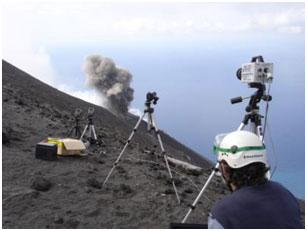 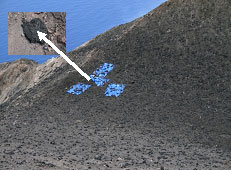
Within CLERVOLC (program 1), the remote sensing group of LMV-OPGC completed a multiparametric experiment at Stromboli volcano during September-October 2012. The aim of the experiment was to:
- Achieve complete characterization of explosive eruption dynamics at the highest possible spatial-temporal resolution;
- Quantify the emitted products; and
- Better understand the processes that control the emission and ascent of a Strombolian eruption plume.
The geophysical array included simultaneous data acquisition by:
- Two thermal infrared cameras. One camera was deployed to track, at 200 Hz, hot gas and particle velocities, plus particle size distributions and mass fluxes, across a narrow window immediately above the vent. A second camera imaged, at 30 Hz, plume ascent and dispersion.
- One Doppler radar, deployed to quantify the mass and ejection velocities of the ballistic bombs and blocks.
- A very high frame rate camera, which acquired images spanning the visible and near-infrared to characterize the highest velocity particles carried by the gas phase.
- Two stereoscopic cameras, used to reconstruct the 3-D trajectories of bombs and constrain their sizes.
- One SO2 camera, to quantify the mass of SO2.
Two thermal infrared radiometers, coupled with a pressure sensor sampled at 50 Hz, were also deployed to obtain thermal time series associated with the emission of pyroclasts, pressure variations, and the depth of the explosions through analysis of the thermal-infrasound time delay. In addition, permanent and temporary broadband seismometers and pressure sensors, through collaboration with M. Ripepe (University of Firenze, Italy), were used to complete the geophysical network.
Ejecta sampling was carried out using the “tarp” collection method (as described in the routine measurements section) to provide particle size distributions of the solid part of the plume, and the vesicularity, crystallinity, chemistry and rheology of the magma residing in the shallow system. A tarp was actually sampled during air fall, so that all particles (lapilli coarse ash and Pele's hair) landing in a well-defined area were collected during a single fall out event.
Quantification of a Strombolian explosion
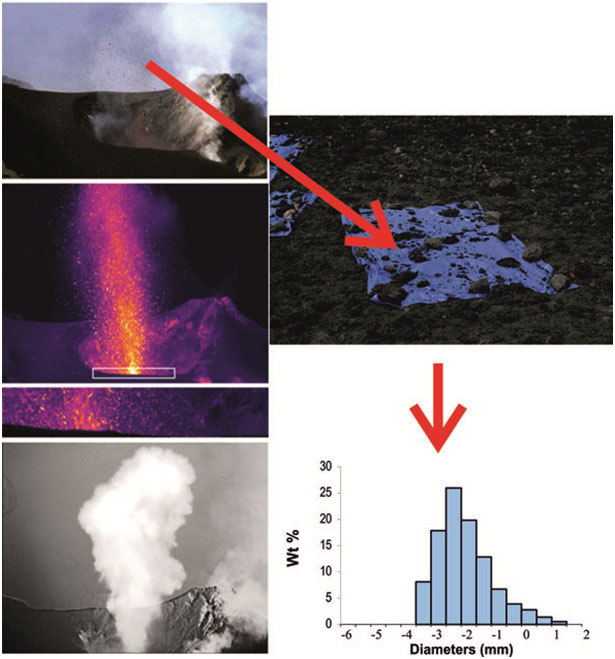
Two types of activity were sampled during the test deployment:
- Type 1 eruptions dominated by gas and coarse (bomb and lapilli sized) ballistic particles; and
- Type 2 eruptions involving a mixture of ballistics and convecting clouds of gas and ash.
Together the physical and geophysical data allowed us to fully constrain:
- Velocities of vent leaving particles and the ascending plumes;
- Ballistic trajectories;
- Shape, size, dimensions and volume of all particles, total volume of bombs and lapilli, and volume flux;
- Bomb and lapilli densities, which were used to convert volumes to masses to obtain magma discharge rate (MDR).
- Event mass and mass flux (and variations in the flux) partitioned between bombs, lapilli and gas.
|