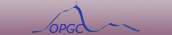 |
|
Recherche |
|
DYNVOLC database
Data currently available : Kilian, Stromboli, Etna, Villarrica, Mauna Ulu
(click on red volcano).
Data available with virtual observatory : Piton de La Fournaise
(click on yellow volcano ).
Take me to the Virtual Observatory portal
|